What is the best way to know how many SSD slots I have?
Do you want to know how many SSD slots your laptop or pc has?
It is always a good idea to have a big storage if you are a video editor or a gamer. However, it is costly, so we buy a laptop or a pc with standard specifications, and we plan to upgrade it in the near future.
However, you should be aware of the number of SSD slots your laptop or PC has before buying so that you can upgrade your storage capacity conveniently in the future.
How many SSD slots does my laptop or PC have?
Currently, the computers we have come with multiple SSD slots. Some of them might have an SSD, but others can be empty. Manufacturers leave them empty on purpose. One can increase the storage size of a PC by adding more SSD.

Ask the manufacturer
The first thing that any wise buyer could do when purchasing a PC is ask for all the necessary specs of the PC. However, this cannot be applied for second hand purchasing as in that case, someone might not get the manual of the PC.
If you are purchasing from Amazon, then you can read about all the features and specs effortlessly.
But what if you don’t have an SSD slot? Then in that case, you will have the HDD slots. These slots can also be used to have the SSD slot.
Checking the motherboard’s specs
Then another thing you can do is to search for the specs of the motherboard. As all the storage devices need a motherboard; therefore, it is important to check the spaces on the motherboard.
Every New motherboard has M.2 slots and Multiple SSD Slots.
Through windows task manager
Now we have to answer the question: how to check SSD slot in laptop without opening ?. The next thing is through the OS. The information in your computer can tell about its specs. All you need to do is follow these simple steps.
- Open the windows task manager.
- Now, in the task manager, open the performance tab, and then open the Disk tab.
- There an interface will open, and you can see the number of slots used.
Opening the PC or Laptop
First, you learned about the number of slots for SSD through specs and manual reading. In case you have lost the manual, and you don’t know about the specs of the motherboard, then it will be better to check and examine the motherboard.
To identify the SSD slots, you must search for some long bar-like structures placed in parallel. These are present on the side of the CPU.
Laptop SSD Slots Checking Without Opening
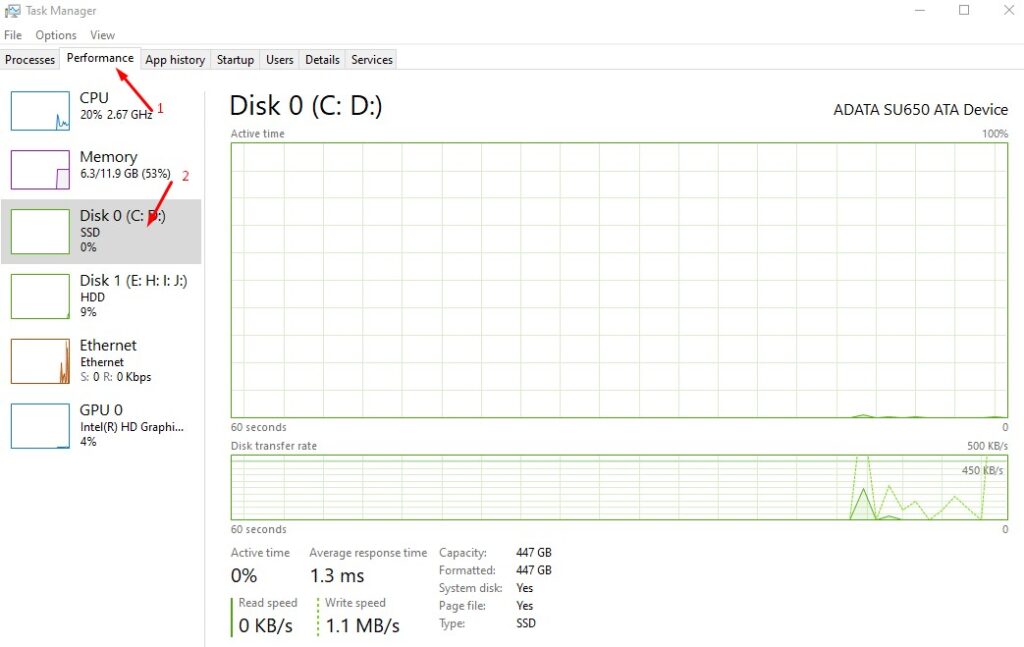
What is an SSD?
Before we initiate, it will be of high value to let our readers know about the SSD.
Just like the storage card of your cell phone, PCs have multiple types of storage device. The SSD stands for solid-state drive, which uses NAND flash memory to store and retrieve data. SSD known for its fast read and write which overall helps to improve computer performance.
It is like a solid disk that stores all the information, be it your images, some software, some files, or whatsoever. There is nothing mechanical in an SSD. It stores data in the form of an electric charge.
Pros of SSD
It is pretty obvious that if we have more storage in our devices, the performance of the device will be affected. A PC is used for many tasks; therefore, it will be great to have more SSD slots.
Now, what will be the real benefits of having more SSD slots?
- The most important thing that we all wish to do through our PC is multi-tasking. We all wish to have a PC that does not stop working when handling different tasks together. If you will use multiple SSDs then it will be easier to multitask.
- Regardless of their number, the SSD will not consume more energy or produce extra heat. Thus, your PC will remain cool.
- Safety of your data is very important. Unlike HDD, SSD is more durable. In case you drop your PC, the SSD slots will stay safe while securing your data.
- You can do your work faster with multiple SSD slots. No need to worry about the lagging and freezing of your system.
Cons of SSD
Fortunately, using multiple SSD is not very harmful. However, there are a few dark points.
- The SSD is way more pricey than HDD.
- The life expectancy of SSDs is pretty low.
- The data lost through SSD is very hard to recover.
Conclusion
Having multiple numbers of SSD slots is very beneficial for multitaskers, and people who need to work only on their PCs. Gamers, video editors, and programmers will love to have such a PC with multiple SSD slots.
- What Laptop Works Best with Cricut? Craft Your Creativity to Perfection - February 22, 2024
- Want to Invert Colors on Your Chromebook? - February 22, 2024
- Your Guide to Choosing the Best Lenovo Chromebook for You - February 21, 2024
Memory is RAM slots not SSD slots.
open Task manager and click on performance and view disk to see what type of storage you have.